Exponential Technologies Across Health Care
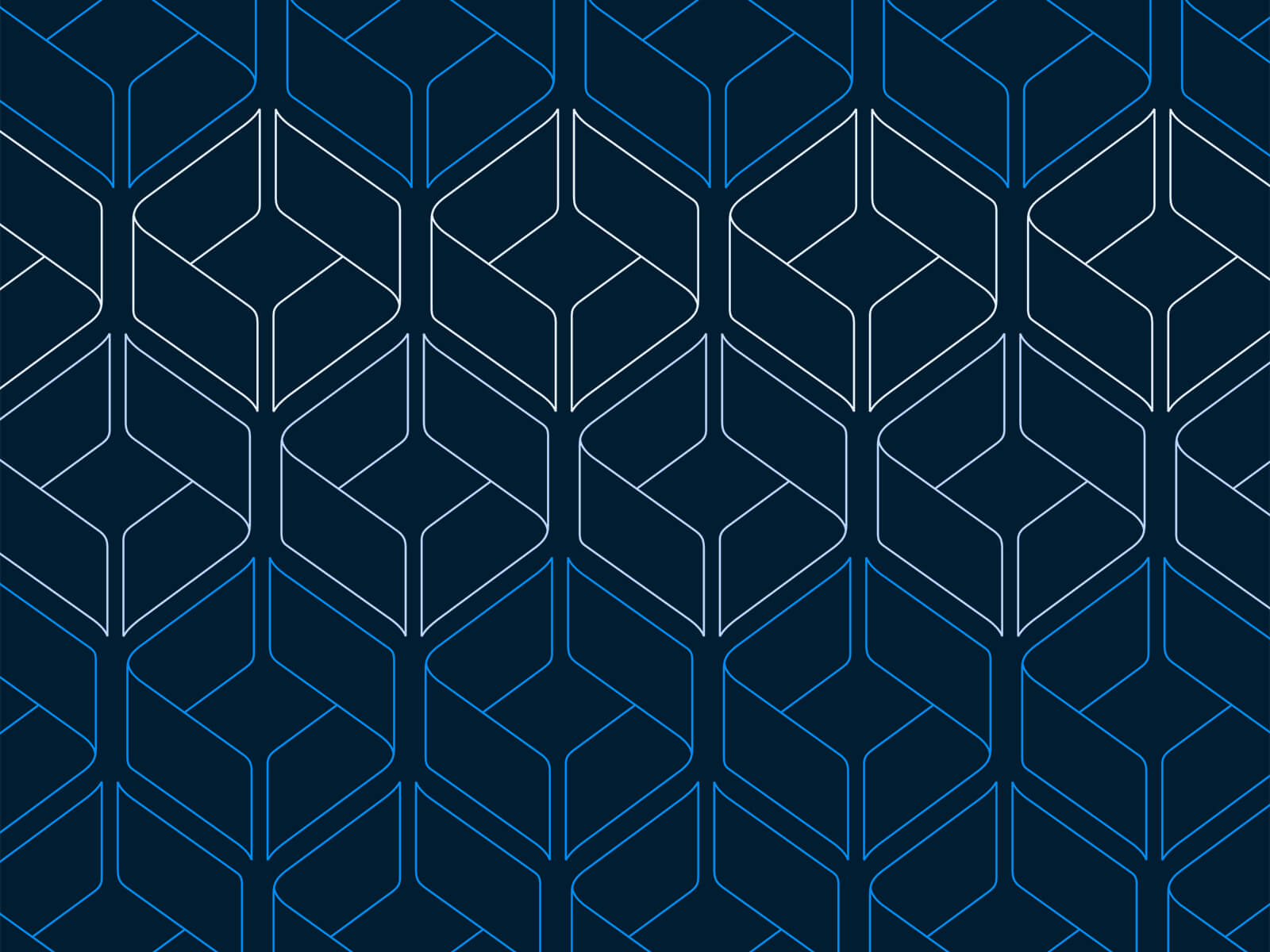
Daniel Kraft, Class 13
In the realm of biomedicine and life sciences, the convergence of ever faster computing, rapidly developing mobile infrastructure, and internet technologies has driven dramatic gains in genomics and high speed sequencing to synthetic biology, molecular diagnostics, bioinformatics, imaging, robotics, artificial intelligence (AI), miniaturization (approaching nano-scale), and the digitalization of medical records. These advances among others, with the overlap of social, mobile, and "game-ification" aspects of health, are leading to the building of novel, rapid growth technologies, platforms, and companies. While IP, regulatory, and reimbursement hurdles definitely remain significant barriers and concerns, the increasing globalization and the rate of technology evolution and innovation are changing the geography and speed of application to the clinic, in broader and more international markets.
For the investor interested in the applications of technologies, appreciating these exponential trends and where they may intersect, and aiming the investment arm toward "where the puck will be" will likely translate to better returns than, for example, backing another "me too" device, pharmaceutical, or diagnostics company. In this article I examine several exponential technology trends in health care and consider how "faster," "smaller," "cheaper," and in some cases "better" tech-nologies—and particularly at their convergence—are changing health care. By recognizing, tracking, and leveraging these trends, new high-yield investment opportunities may become available.
Understanding and Leveraging Exponentials
As a physician–scientist, entrepreneur, and Kauffman Fellow, I've had a relatively unique purview to delve into rapidly developing technologies in my role as faculty, chairing the Medicine track for Singularity University,1 a new and unique educational institution based at NASA-Ames Research Park in the heart of Silicon Valley. Singularity University was co-founded by Ray Kurzweil, the well known inventor (text to speech, Kurweil synthesizer, etc.) and author (Singularity Is Near2), and Peter Diamandis, physician–founder and Chairman of the X-Prize, which is most notable for the Ansari X-prize won by Burt Rutan and his team with Space Ship One in 2004 (now being commercialized by Virgin Galactic and often credited with jump-starting the commercial space industry), as well as for the Progressive Automotive X-prize, ongoing Archon Genomics X-prizes, a new Tricorder X-prize, and others.
In Summer 2012, Singularity University will run our third 10-week graduate studies program, bringing together 80 students from over 35 countries, together with a who's-who faculty ranging from technologists like Larry Page and Vint Cert, to Dean Kamen, to those in venture (Steve Jurvetson, David Bloom). We have also run quarterly 5-7 day executive programs targeted for C-level executives, investors, entrepreneurs, and government officials to dive into and cross-train in rapidly developing technologies in our eight core tracks (Medicine, Biotechnology, Robotics, Artificial Intelligence, Nanotech, Space, Energy, IT, and Computing, with overlaying tracks of Policy, Law and Ethics, and Entrepreneurship). Multiple funded companies have already spun out from Singularity University, ranging from GetAround (future of transportation focus with a peer–peer car sharing, already on the market and winner of the 2011 TechCrunch best new company award) to CiviGuard (leveraging mobile for disaster response) and ACASA3 (3D house printing).I recently chaired a 6-day executive program at NASA-Ames called FutureMed, where we brought together thought leaders across multiple fields to address how exponential technologies are and can—especially at their convergence—be leveraged to positively impact health and medicine. This potential and need is particularly relevant given our current era that includes a burgeoning aging population (10,000 baby boomers turn 65 each day in the United States), cost constraints, and an obesity epidemic.
Exponentials in Health Care: Faster
Human minds tend to think linearly, and we often fail to grasp the power of exponentials intuitively. If I take 30 linear steps, I get 30 paces—30 exponential steps, and my last step spans more than a billion of my original step. Or, if a lily pad doubles each day—2…4…8…16…32…64—by day 15 (215) there are 32,768 but only 15 days later, by day 30 (230) we'd be left with just over 1 billion lily pads.Nowhere has the power of exponential growth been more evident than in the inexorable progress of "Moore's Law"4—as predicted in 1965, computational speeds double on an annual basis (on average, and this despite wars, recessions, and economic downturns).
In health care, the impact of incredible computational clock-speed in exponentially smaller spaces is most evident in the realm of medical imaging. The state-of-the-art CT scan of the late 1990s had a resolution of ~100 slices (5122 pixels) and the 50MB data could be contained in about 50 telephone books; today, rapid CT scans completed in 30 seconds have increased to a resolution of 2400 slices, 20GB per scan or 800 books, and within five years CT scanners will regularly obtain a Terrabyte (800,000 books) per scan. We are now in the era of real-time functional MRI (fMRI), enabling researchers and new companies (like Omneuron) to delve into the brain and address issues, personalize drug regimens, enable real-time biofeedback, and show efficacy in the treatment of ailments from chronic pain to addiction.Imaging technologies are converging, such as the combination of two modalities (positron emission tomography with CT Scan: PET-CT), and the addition of molecular imaging has enhanced, for example, the location and qualities of a particular tumor as pioneered by Stanford University and others.5 Increasingly improved CT scan and layered MRI reconstruction enables a resolution, not only for diagnosis but (in the case of electron microscopy) also down to the neuronal level, for understanding of normal and diseased pathways and insights into possibilities for reverse engineering neural circuitry. This convergence has led to far higher levels of precision, but also presents a challenge in terms of the ability to store, read, and interpret the images and extract in a useful way the massive amount of digitized data contained within.In addition, scanners are getting smaller and are more mobile. For example, relatively portable CT6 and MRI scanners down to hand-held ultrasounds (at less than a $5K price point7) are now entering the clinical arena.The speed, resolution, and access to imaging data are now enabling the redesign of major traditional medical procedures. For example, screening colonoscopies (due for everyone at age 50) are a major tool in the detection and prevention of colon cancer (which kills 50,000 U.S. residents each year8—many of whom skipped the screening). In many cases, screening colonoscopies will be supplanted by "virtual colonoscopy," a fast CT scan followed by 3D reconstruction of the patient's colon and a virtual "fly through" by the radiologist. With the addition of artificial intelligence (AI) algorithms, a higher degree of sensitivity and specificity is being gained for the detection of pathologic lesions that may have been missed by standard radiologist reading.9 Such cloud-based computational and AI-enhanced techniques are increasingly employed in the screening of mammograms and pap smears, and similar AI-enhanced "readings" will likely supplant the standard pattern-recognition skills of the dermatologist in many settings, enabled by mobile, cloud-based image detection and analysis.A Stanford University spinout called HeartFlow is leveraging image data through high-speed CT scan and cloud-based data analysis. Using computationally intensive cloud-based fluid dynamic calculations, their technology can measure and indicate to the cardiologist the rate at which blood is flowing before and after a coronary artery lesion,10 and determine which patients require invasive cardiac-diagnostic procedures (including placement of coronary stents) and which patients do not. This technology has the potential to save money, prevent almost half of the unnecessary angiograms performed each year, and improve patient outcomes at lower overall cost.11
The Digitization of Medicine
With dramatic increases in computational speed as well as the speed and ubiquity of low-cost wireless data transmission and ever smaller storage space, the era of the mobile media device has quickly emerged as a force in health and medicine. With the advent of "apps" there are now over 17,000 health- and medical-related applications for the iPhone and Android platforms alone. These apps span the range from fitness (e.g., Nike and RunKeeper, personal trainer programs) to disease-specific (e.g., enabling diabetics to track blood sugars, diet, and activity) and mobile drug reminder/ compliance programs, to a widening array of educational/training and the now-ubiquitous clinician resources such as ePocrates (recent IPO-EPOC), which incorporates drug pharmarcopia and other information of use to the clinician at the point of care.
Indeed, an increasing number of physicians are dependant on mobile applications not only to access medical information and databases, but also to track individual patients via their electronic health record (EHR), including lab and radiology results. Now, for example, clinical data can even be streamed directly from the inpatient ward or ICU,12 and the FDA has approved the first iPad applications enabling radiologists to do formal readings (even from the golf course).13IBM's Watson Artificial Intelligence system has demonstrated recently its ability to beat Jeopardy champions, and the power of this AI is now being turned to medicine.14 More primative versions of "narrow" AI are already in our hands, whether for helping pharmacists prevent drug–drug interactions or for the physician in interpreting EKGs. Handheld accessible portals will soon allow tethering to the power of a cloud-based "Watson" as applied to health and medicine, enabling the clinician to enhance the speed, accuracy, and cost-efficiency of diagnostics; obtain decision support for applying the increasingly available evidence-based data sets; and choose the most appropriate therapy for an individual patient (i.e., drug, device, or surgical intervention).As we move further from the era of paper-based medical records (less than 20 percent of U.S. medical records are currently electronic)15 toward electronic medical records (EMR), the data explosion will further accelerate. With this growth come new opportunities to leverage this "big data" for enhancing patient care and lowering costs. As an example, I have turned in my traditional stethoscope for a digital one that can record, analyze, and synch my cardiac examinations with a mobile phone app.This transformation of analog to data, and the speed and access to data—whether in the form of a radiologic exam, EMR, or handheld device—are ushering in a new age of digital medicine at the intersection of biomedicine, IT, health data, wireless, and mobile. This convergence has profound implications for the future of health care. Medicine will transform from an "art" traditionally bound to the clinic or hospital, to one driven by numbers and quantification as well as crowd-sourced, in the home and mobile on the patient's body.
Exponentially Distributed Health Care
Traditional medical care has occurred in the physician's office/clinic or hospital, with the days of "house calls" by a primary care provider now only a distant memory. As technology trends advance in terms of bandwidth and resolution, however, the ubiquity of web-based communication (from email to video-interaction with the healthcare provider) means that doctor–patient communication is going virtual and moving back to the patient and their home. From web-based portals such as AmericanWell.com that enable HIPAA compliant (and reimbursable) Skype-like interactions between patient and physician, to a widening array of web-integrated wireless scales, blood pressure cuffs, and monitoring devices, the time traditionally taken out of work—including travel, waiting room time, and other inconveniences—can be supplanted by telemedicine-enabled clinical visits. The majority of patient–doctor interactions do not require the "laying on of hands" or a physical exam, and can be effectively replaced by tele-visits. Cisco, for example, has developed a HealthPresence system leveraging their experience in high-end corporate teleconference systems and infrastructure for medicine integrated with digital stethoscopes, otoscopes, and other enabled clinical tools to facilitate physical exams at a distance (usually facilitated by an attendant). Clinical outcome studies demonstrate parity in clinical outcomes and patient satisfaction with traditional in-person clinical visits.16
In some cases a specialist could visit via telepresence-enabled robotics. The PR-2 robot (InTouch Health17), for example, is designed to enable a clinician to autonomously move and interact in a clinical setting, and in such a way a clinician can visit a remote hospital and provide guidance and interaction and even limited examinations. These sorts of telepresent and technology-enabled interactions have significant potential value for application in remote and underserved areas where fully qualified clinicians positions are difficult to staff, and could be used to augment community health workers, for example.A related arena further enabling patient–physician interaction is the advent of personal monitoring devices, which are going wireless with the potential for integration into the patient's personal or electronic health record. With an increasingly capable array of internet-enabled glucometers, scales, blood pressure cuffs, pedometers, and sleep and cardiac monitors, data can be obtained far less expensively and more conveniently than in the standard clinical setting. The advent of the "Quantified Self30" movement is leading to the inspiration and commercialization of devices such as the FitBit (a web-enabled pedometer) and the Zeo sleep monitor. These consumer-based tools are increasingly integrated with social media (enabling users to share their steps, sleep "score," weight, etc.), and beyond fitness and wellness applications have definite applications in the tracking and treatment of chronic disease.While emerging technologies are enabling the traditional patient–doctor relationship and encounter, they are "super-enabling" the surgeon. Robotic surgery as pioneered by companies such as Intuitive Surgical is now well established, with the number of robotically enabled surgical cases increasing over 3-fold since 2006.18 Various fast paced technology fields are currently being merged on top of robotically enhanced surgery platforms. These include the layering of radiologic information or "enhanced reality," which will enable the surgeon safer identification of structures (tumors, blood vessels, etc.) while visualizing the surgical field through 3D goggles. Tele-mentoring, in which a remote surgeon can help guide an on-site surgeon, is being tested. As robotic surgery progresses and the user interface and end devices become more facile and capable, the field of "scarless" surgery19 is developing, in which a surgical robot enters the abdomen via the esophagus and stomach rather than skin incisions, leaving no external scars.Surgical robotics today are non-autonomous, that is, they require directed surgeon hand-to-controller input for every suture and step. Augmented manual control for the surgeon, and more importantly for the disabled (i.e., quadriplegics), is being developed in the field of Brain Computer Interface (BCI). Postage-size microarrays, placed on the motor cortex of spinal cord-injured patients, integrated with signal processing, enable the paralyzed to control a cursor, wheelchair, or robotic limb.21 As bionic/robotic prosthetics improve—as epitomized by the lightweight Luke-arm prosthesis22 developed by Dean Kamen and his team at DEKA with 17 degrees of movement—it becomes conceivable for those with amputations or spinal cord injuries to achieve some degree of autonomous function. For those with paralysis or motor weakness, or those seeking "enhancement" of motor strength, wearable robotic-like bionic exoskeletons enabling paraplegics to walk have just entered clinical trial use (Berkeley Bionics' eLegs). These advances will likely not only change the way those with strokes, paralysis, and generalized weakness ambulate but also enhance the normally-abled (e.g., the soldier carrying over 100lbs of gear, or nurses who can use such technology to more easily move patients).
Exponentially Smaller
As memory chips, batteries, and motor components continue to shrink in scale and price point, medical technology has become ever smaller and more capable. Amazing things are in development, such as integrated, swallowable pills (the iPill)23 now on the market that can image, telemeter video, and release drugs. Also in development are sub-centimeter micro-bots that can travel through various structures and may be able to provide diagnostic and therapeutic actions.24 Multiple swallowable-sized bots could potentially self-assemble in the stomach and have further functionality.
In another example, pacemakers continue to miniaturize, with Medtronic recently announcing a new self-contained cardiac pacer that does not require a pacing wire and can be implanted by a minimally trained clinician directly into the heart wall. This device can synch with a local mobile phone via Bluetooth type technology to enhance monitoring and tuning.25In the laboratory and diagnostic arena, the era of microfluidics (in which liquids ranging from blood to chemicals are handled at the microliter scale) is supplanting standard large-bench labs and enabling hundreds to thousands of tests to be performed at a fraction of the price, on chip-based platforms.26 Microfluidic-based genetic analysis (Fluidigm—FDGM) diagnostics can be utilized at the point of care by minimally trained staff and will extend the capabilities of first world labs, but especially of those in remote areas and less developed countries.Further down the rabbit hole is the evolution of nanotechnology, which is essentially design at the molecular level. This development holds promise for the eventual construction of nano-scale structures ranging from artificial red blood cells to nano-robots that can autonomously perform diagnostic or therapeutic functions.
Exponentially Cheaper
Lower cost is not something normally associated with health care, but in some arenas such as genomic sequencing, the cost and speed are dropping at a greater-than-exponential rate. At the turn of the millennium, the first human genome was sequenced at a cost of millions, in a project that ran over several years. Until recently, the cost of a sequence was over $100,000; this number has dropped to approximately $10,000 in 2010 and is projected to fall to the sub-$1,000 level within two years.27 Some predict (e.g., Eric Shadt, CSO of Pacific Biosciences) that within five years genomes may be essentially "free"—and similar to mobile phone plans, the patient (or client) pays a subscription to have access to analysis of their data. Traditional pharmaceutical companies, for example, may subsidize such testing as the movement toward personalizing therapies and prevention evolves. "Big Data" and crowd-sourced, familiar genome analysis is expected to unlock much of the potential and promise of clinical translation of genomic sequencing.
Today for ~$200 through companies like 23andMe and Navigenics, individuals can already gain access to much of their genetic information via Single Nucleotide Polymorphism (SNP) analysis. While direct-to-consumer genetic testing remains somewhat controversial, actionable information (e.g., data on genetic predispositions that may spur lifestyle modification or heightened vigilance/screening, or basic pharmacogenomic information on genes that affect drug metabolism) can potentially inform more intelligent and patient-specific dosing and drug selection.Today we live in an era of "one size fits all" medicine, with many drugs not working on 50 percent or more of the population—and worse, having some degree of toxicity without benefit in others. As individuals' genomic data becomes more ubiquitous and available as a commodity at low cost, the value propositions can facilitate the long promised era of "personalized medicine" in which the goal is to select the right drug (and dose) for the right patient, given at the right time.Many quantifiable aspects of each individual patient are becoming dramatically more available at lower price points, including proteomics—the measurement and analysis of the thousands of proteins from the blood (or other clinical samples) that can be measured and potentially can lead to early disease detection or tailoring of therapy. Several groups are working on point-of-care devices to measure blood-based proteomes.Proteomic data woven together with genomic, environmental, and other personalized data has the potential to significantly change the practice of medicine—integrated in the new fields of systems biology and systems medicine. Lee Hood, the physician–scientist who founded the systems biology field,28 summarizes its potential with the term P4-Medicine, or medicine that is Predictive (genes), Preventative (take steps based upon predictive risk), Personalized (the prevention is personalized), and Participatory. Participatory refers to the sharing of individual data (minding privacy concerns), enabling data mining across similar and dissimilar patients, as well as the potential for individuals to connect with others who share common genes or conditions. Early versions of participatory medicine platforms include PatientsLikeMe.com and CureTogether.com, which enable motivated patients and their families to share their clinical information, as well as compare notes on different therapies.
Looking Ahead: Exponentially Improved Medicine
No matter how advanced, medical technologies will not be adopted unless they truly improve care and—of increasing importance—lower costs. As an example, personalized oncology will leverage the exponential technology trends in genomics, proteomics, and bioinformatics to enable the oncologist to identify chemotherapies and targeted drugs and cocktails that are a true fit to the individual's particular cancer.
In the field of regenerative medicine (the repair and regeneration of tissues damaged by aging, disease, or trauma), the controversy around embryonic stem cells is being circumvented by the advent of induced pluripotent stem cells (iPS). iPS cells can be derived by reprogramming an individual's skin or other cells, and eventually through integration with technologies such as 3D printing,29 will lead to an era of personalized regenerative medicine. As technology advances, doctors will gain the ability to 3D-generate functional, implantable organs derived with cells from the same patient—requiring no immunosuppression or risk of rejection.As this article has briefly described, new opportunities and capabilities emerge in this new era of personalization, rapid innovation, and the convergent integration of game-changing exponential technologies. For a more dynamic and visual presentation of these ideas, a TEDx talk I gave on April 6 summarizes much of this in 18 minutes and is available on YouTube.30Through appreciation of the power, speed, and direction of accelerating exponential technologies that can be applied across multiple realms of health care, the investor (in traditional life science, and increasingly in IT, pharma, or medical devices) may be able to identify and place their bets on "where the puck is going," rather than where it has been. The returns, while perhaps not exponential, are likely to outsize those made on more static or linear technology-based opportunities.

Daniel Kraft
A partner at Proteus Venture Partners, Daniel is a Stanford- and Harvard-trained physician, board certified in Internal Medicine and Pediatrics and fellowship trained in hematology/oncology with extensive research in regenerative medicine. Faculty positions at Stanford and UCSF allowed multiple publications and patents, and he is the inventor of the MarrowMiner (being commercialized for bone marrow transplantation) and founder of RegenMed Systems developing novel tools for regenerative medicine. He is also flight surgeon with an F-16 squadron in the California Air National Guard, chair of the Medicine track for Singularity University, and director of its FutureMed executive program. Daniel has spoken at conferences including TED, TEDMED, TEDx, and Web2.0. He recently founded IntelliMedicine, focused on leveraging exponential technologies to enable evidence-based, effective personalized medicine.
1 http://singularityu.org/. While the term singularity and some of Ray Kurzweil's writings and predictions are somewhat controversial, the focus of Singularity University is around understanding, cross-training, and leveraging technologies to address major global challenges.2 Raymond Kurzeil, The Singularity Is Near: When Humans Transcend Biology (New York: Viking Press, 2005).3 See Green Initiatives, "GSP-09 Team Project: ACASA."4 Intel Corporation, "Moore's Law, 2006."5 See Molecular Imaging Program at Stanford (MIPS); Ralph Weissleder and others, Molecular Imaging: Principles and Practice (Shelton, CT: People's Medical Publishing House, 2010).6 See NeuroLogica portable CT.7 e.g., the Personal Ultrsound from Signostics Medical.8 B. K. Edwards and others, "Annual Report to the Nation on the Status of Cancer, 1975–2006, Featuring Colorectal Cancer Trends and Impact of Interventions (Risk Factors, Screening, and Treatment) to Reduce Future Rates," Cancer 116, no. 3 (2010): "Discussion" para. 5, doi: 10.1002/cncr.24760.9 Danielle Roxanne Ouhadi and others, "Virtual Dissection CT Colonography: Evaluation of Learning Curves and Reading Times with and without Computer-Aided Detection," Radiology248, no. 3 (2008): 860–68, doi: 10.1148/radiol.2482070895.10 Caitlin E. Cox, "DISCOVER-FLOW: CT-Derived FFR Measurements Show High Diagnostic Accuracy."11 Nick Veronin, "New Heart Care Technology Getting Trial at El Camino: Fluid Dynamics Equations Tell Doctors Whether Invasive Procedures are Warranted."12 See AirStrip Technologies.13 See the Mobile MIM, MIM Software.14 Dan Nosowitz, "IBM's Watson Makes the Move from Answering Trivia Questions to Making Medical Diagnoses."15 Andrew Clark, "The Carrot and the Stick of EMR Adoption," Leavitt Partners Blog, para. 9.16 See home telemedicine studies gathered by the Scottish Center for Telehealth.17 InTouch Health, "World's First Remote Presence Robot Used by Healthcare Experts in Elder Care Begins Clinical Testing [press release]."18 Danil V. Makarov and others, "The Association Between Diffusion of the Surgical Robot and Radical Prostatectomy Rates," Medical Care 49, no. 4 (2011): 333–39.19 NOTES, or Natural Orifice Translumeal Endoscopic Surgery.20 See the Brown University-sponsored organization Brain Gate.22 See Sarah Adee, "Dean Kamen's 'Luke Arm' Prosthesis Readies for Clinical Trials."23 See Erica Naone, "Philips iPill."24 See Michael and MedGadget, "ViRob: A Cavities Crawler."25 Emily Singer, "A Pacemaker the Size of a Tic Tac: Medtronic is Using Microelectronics to Make a Pacemaker so Small it Can be Injected."26 See the Quake Group website, Stanford University Department of Bioengineering (2005).27 Kris A. Wetterstrand, "DNA Sequencing Costs: Data from the NHGRI Large-Scale Genome Sequencing Program."28 See Institute for Systems Biology, 2011.29 See Organovo, "Tissue on Demand," 2010.30 Daniel Kraft, "What's Next in Health Care?"[video], TEDx Talks.
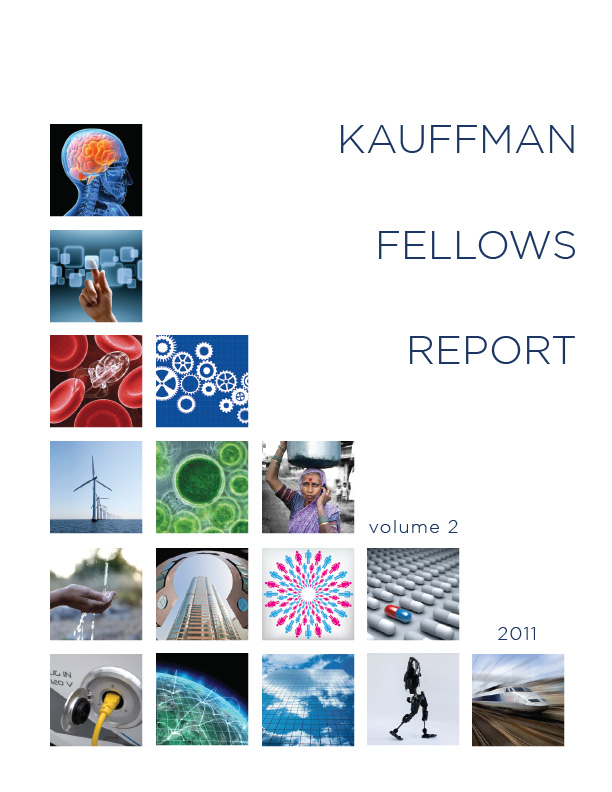